Different kinds of vibrations in electrical machines
Hello again! Have you seen the earlier post about magnetomechanical analysis? If not, I recommend you at least open up the link in a new tab, to read after this post. After all, that one went quite deep into the magnetomechanical interaction on the material level. By contrast, this post is mostly about large-scale magnetomechanics.
Specifically, I’m gonna be writing briefly about the different kind of mechanical phenomena and vibrations in electrical machines.
Background
This Autumn, I’m co-lecturing a course on mechanics of electrical machines. So, that’s one of the main reasons why I’ve been going on about that stuff recently.
The second teacher of the course is one Mr. Timo Holopainen (D.Sc.) from ABB Finland. He definitely knows his stuff (check out his thesis for a small example), so I’m going to follow his classification of the phenomena, almost to a T.
Mechanics and vibrations in electrical machines
Long story short, mechanics of electrical machines can be divided into different subcategories. Here’s one division, on a decreasing (typically) order of importance.
0. Rotation
This is the “duh” category, being so obvious that it hardly deserves a mention. But it is the most important kind, and has the strongest coupling between magnetics and mechanics. The entire concept of an electrical machine is based on it, after all.
1. Torsional vibrations
Alright, let’s move on to more interesting topics. Torsional vibrations can be downright nasty. As their name suggests, they refer to vibrations around the shaft.
For example, let’s say we have a machine driving a heavy load. Imagine that, right? Between the machine and the load is a shaft. And, since the machine is producing some torque that the load is “consuming”, the shaft is twisted around its axis a little. It’s not infinitely stiff, after all.
This situation is illustrated in the figure below. One of the rotating masses is the machine, while the other one is the load. The twist angle is then of course .

Ideally, this twist angle stays constant, and the speed of the machine (or load) stay constant as well. In practice, however, neither the electric nor the load torque are perfectly smooth – there’s some ripple. And this ripple can cause small variations in the torsion angle of the shaft, too. Correspondingly, the rotation speeds of both the machine and load oscillate around their mean values.
These torsional vibrations can be quite insidious. They rarely produce much noise, nor produce tangible vibrations in the machine frame for instance. Often, the first indication of a torsional instability problem is the shaft or something connected to it breaking down due to fatigue.
Torsional vibrations are essentially system-level vibrations. Meaning, their presence or absence cannot be predicted based on machine data alone. Instead, the entire drivetrain has to be considered, to see if there are any potentially harmful resonance modes. And in other words, the exact same machine may perform just fine with certain loads, but suffer a shaft failure with others.
In torsional vibrations, the coupling between magnetics and mechanics is very strong. After all, the torque produced by an electrical machine is intimately coupled to the angle of the rotor. Hence, oscillations in said angle result in oscillations in the driving torque. Depending on the case, these oscillations may dampen or feed the mechanical vibrations, in the worst case resulting in an unstable system.
2. Lateral vibrations
Torsional vibrations are not the only mechanical issue affecting the rotor. It can also bend around the x- and y-axes, forming a banana shape for example. These are called lateral vibrations, or bending vibrations.
Also these vibrations are very much magnetomechanical in nature. Since the rotor is, well, rotating, any mass inbalance requires a radial counter-force, lest the rotor be bent off-axis. In a good day, the bending stiffness of the rotor plus shaft provides this force, and all we have to suffer is a very slightly banana-shaped rotor plus some vibrations and bearing forces.
As the rotor-banana is also rotating, we say it’s whirling.
However, if the rotation speed is too close to one of the bending natural frequencies of the rotor assembly, bad stuff happens. The mechanical damping in the system is almost negligible – we are speaking about solid steel after all. Thus, even a small excitation can result in large vibrations. For this reason, very long machines such as turbogenerators have very narrow allowable speed ranges. The same applies to high-speed machines, which are generally short but rotate very fast.
And that was the mechanics part only. Magnetics come into play when we realize that the rotor bending shortens the airgap on one side, and lengthens it on the opposite. As a result, the air-gap flux density becomes nonsymmetric, and a net force starts to act on the rotor.
In the simplest case, this force is pulling towards the shortest air-gap, trying to displace the rotor even more.
However, things get more complex if we have anything in parallel – like a rotor cage or damper winding, or parallel paths in the stator.
In that case, the un-even air-gap flux can induce circulating currents in the parallel winding parts. These currents, naturally, oppose the inducing flux wave, typically decreasing the unbalancing force.
However, the exact dynamics are quite complex to say the least. You see, the rotor position affects the flux density, which affects the induced currents (with a delay thanks to the inductances and resistances of the winding), which affect the forces, which affect the rotor position. So we have a nice, complex feedback loop going on.
Thus, what actually will happen is very much problem-dependent. But the point is, even here the interaction between mechanics and magnetics is quite significant.
3. Stator vibrations
These are exactly what their name suggests – vibrations in the stator only. As a recap, below you can find the first two natural modes of a 37 kW induction motor. The first is roughly oval-shaped, while the second one resembles a triangle.
As the modes pictured have resonance frequencies of roughly 1000 Hz and 3000 Hz, you can probably guess the main hazard of stator vibrations.
Exactly – noise. They can produce lots of noise.
Unlike the previous types of vibration, the magnetics of stator vibrations are often largely uncoupled from the vibrations. Or, to be more exact, the feedback from the vibrations back to the magnetics is often quite minor. The displacements are far too small to affect the geometry of the magnetic circuit. Furthermore, the effect of the associated stresses on the permeability is typically rather small when the entire machine is considered.
Note, however, that I didn’t say that the feedback didn’t exist – only that it’s typically small. Some particular machines might be an exception to that rule.
Furthermore, the stator vibrations are of course excited by magnetic forces. So, the feedforward from magnetics to mechanics is indeed strong. The magnetic forces acting over the airgap usually dominate, but magnetostriction inside the iron can also contribute. So, some advanced material modelling may be in order.
Conclusion
The three (usually) most important types of vibrations in electrical machines are:
- Torsional vibrations: system-level with strong magnetomechanical interaction and plant-destroying capacity
- Lateral vibrations: machine-level with strong magnetomechanical interaction and machine-destroying capacity
- Stator vibrations: stator-only vibrations with typically weak interaction, and eardrum-destroying capacity only
ADDENDUM: Are your mechanics skills rusty?
Want to learn more about mechanics, and finite element analysis especially? Well, you’re in luck as my friend Łukasz just what you need.
Meaning, an awesome course on the basics of FEA in mechanics. Łukasz has a very practice-oriented approach, so he won’t be boring/confusing you with too much mathematics. Even more importantly, his decade-long experience really shows.
At the moment of me writing this, the course discount is still on, meaning it’s priced at roughly two typical textbooks. Or a couple of pub nights. Or one, if you’re the Mountain, or are a person of very refined taste. Besides, you should get your employer to pay for the course – they’re spending more than that on toilet paper each week.
So, take the course and save yourself lots of time in the almost-immediate future. And frustration. Especially frustration – stress kills, after all.
By the way: please tell Łukasz that Antti sent you, and I’ll get a small commission. Or don’t – it’s a good course no matter what.
Check out EMDtool - Electric Motor Design toolbox for Matlab.
Need help with electric motor design or design software? Let's get in touch - satisfaction guaranteed!
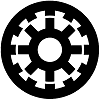